This fact sheet supports the ‘Opportunities for soil carbon management in Central West NSW’ webinar, delivered as part of the ‘Soil carbon capacity building resources for farmers and advisors’ project, proudly supported by the NSW Department of Climate Change, Energy, the Environment and Water (DCCEEW).
In this webinar, Dr Abe Gibson, Soil Scientist and Research Fellow (Southern Cross University) and Dr Cassandra Schefe, Soil Scientist (AgriSci) explore:
- what soil carbon levels can be expected in Central West NSW
- what factors influence soil carbon
- what management practices can be used to increase soil carbon levels.
Soil carbon plays a crucial role in both the environmental sustainability and economic viability of agriculture in Central West NSW. The region’s fertile soils are integral to farming and grazing, and the opportunity to enhance soil carbon presents multiple benefits.
Key messages
- Most soil carbon is derived from the capture of atmospheric CO2 into plants, converted into plant material.
- All soils have a threshold for the maximum amount of soil carbon storage (ceiling), based on climate, soil type and management.
- Soil carbon values will fluctuate over time based on rainfall, biomass input and soil management.
- Build organic matter and soil carbon by fixing soil constraints and increasing soil fertility for optimum plant growth.
- Agricultural systems depend on the ongoing decomposition of organic matter to release nutrients necessary for plant growth, such as nitrogen through mineralisation. Due to the dynamic nature of these systems, maintaining stable soil carbon levels over time requires continuous inputs of carbon and nutrients. Consequently, increasing soil carbon levels requires additional inputs, which may result in extra costs.
- New research in the Central West region is demonstrating benefits to soil health through increased plant diversity in cropping rotations, which may slowly increase soil carbon values over time.
Importance of soil carbon
- Soil organic carbon can improve soil fertility, water retention, structure, and aeration, boosting crop and pasture productivity.
- Enhanced soil fertility supports better root growth and microbial activity, essential for healthy farming systems and building soil carbon.
- Higher carbon levels improve the soil’s ability to withstand extreme weather events, such as droughts and floods, which are increasingly common in the region.
- Increasing soil carbon is a key strategy in mitigating climate change by capturing and storing atmospheric CO₂.
- Practices that sequester carbon or promote soil health are important to demonstrate environmental stewardship. Demonstrating this stewardship will become increasingly important for maintaining market access into the future. Recognising this should be an industry priority.
Factors that affect potential soil carbon values
Rainfall – a key driver of soil carbon is rainfall, as rainfall dictates plant growth.
- High rainfall – greater plant growth, increased biomass, which can be decomposed into organic matter.
- Low rainfall or drought years – low biomass inputs results in depletion of organic matter and soil carbon.
- Dry conditions result in lower microbial respiration of ‘old’ soil organic carbon, so dry conditions can preserve soil organic carbon.
Soil type and condition
- Clay soils can ‘hold’ more carbon as reactive minerals protect carbon from microbial accessibility, directly and via aggregation.
- Low pH soils build less carbon, due to impaired microbial function and plant growth.
- Crusted, dispersive or compacted soils will require amelioration to build more carbon.
- Each soil has a threshold carbon capacity, not an ongoing linear increase.
- Address key limitations before focussing on soil carbon accrual.
Managing soil constraints for soil carbon success
Soil pH – soil acidity is generally the most limiting factor in plant growth in productive farming systems:
- Aluminium toxicity occurs in soils which contain aluminium and are strongly acidic (pH <5). Likely soil types with aluminium include ironstone rich soil and soil with the dominant clay mineral kaolinite. Generally, correcting soil acidity will alleviate toxicity symptoms. Tolerance to aluminium varies amongst plant species:
Tolerance | Species | Critical Values Aluminium |
---|---|---|
Highly sensitive | Lucerne, barley, medics and canola | <5 mg/kg |
Sensitive | Red clover, sensitive wheat, phalaris | 5-10 mg/kg |
Moderately tolerant | Rose clover, tolerant wheat, ryegrass, vetch | 10-20 mg/kg |
Highly tolerant | Lupins, oats, triticale, sugarcane | 20-30 mg/kg |
Source: Peverill, K.I., Sparrow, L.A., & Reuter, D.J. (1999). Soil Analysis an Interpretation Manual.
- Reduced nutrient availability and plant response to applied nutrients.
- Reduced microbial function.
- Poor rhizobium inoculation of legumes, poor persistence of lucerne stands.
Soil pH can be increased through liming. Soil test soil to determine lime requirements and to ensure that other soil conditions aren’t limiting plant growth, such as:
- Soil sodicity – may occur through the region, resulting in poor soil structure, water infiltration and plant establishment.
- Salinity – decreases the ability of the plant to take up soil water, resulting in poor vigour and persistence of preferred species.
- Compaction – reduces the ability of plant roots to penetrate the soil to access water and nutrients.
- Waterlogging/drainage – reduces oxygen availability to roots and microbes, shutting down plant growth and microbial function (g. nitrification).
- Nutritional deficiencies/toxicities – will reduce plant vigour and competitiveness.
Optimising plant growth and microbial function increases the ability to hold and build carbon through greater organic matter input and cycling.
Management practices that contribute to soil carbon
Biomass input
- Legume-based pastures and pulses (biomass and organic nitrogen).
- Crop residues retained.
- Groundcover maintained.
Soil constraint amelioration (e.g. liming) = growing the best plant possible
System fertility – apply adequate nutrients.
- 1,600 soil samples in southern NSW cropping soils have found soils with high soil carbon + good pH status (pH >5) + legume history = good yields.
- Range of soil carbon values from 0.7-4.5% in cropping systems.
- CWFS region soil carbon values are 0.3-2.5% – higher values may be achieved.
Local plant diversity research on soil carbon
CWFS hosts a Soil CRC project, ‘Plant-based solutions to improve soil performance’, trialling a range of summer cover crop, intercropping and temporary intercropping treatments. The field trial is monitored for biomass production and grain yield, soil water use, soil health and changes in soil organic carbon.
Trial results indicate that building soil carbon using these summer cover crop, intercropping and temporary intercropping treatments will be a slow process. While an increase in total soil carbon has not been measured from the research, there has been a positive response in early soil health indicators and reduced disease loads in the diverse systems trialled. Integrating summer cover crop, intercropping and temporary intercropping treatments should be considered as part of a planned rotation.
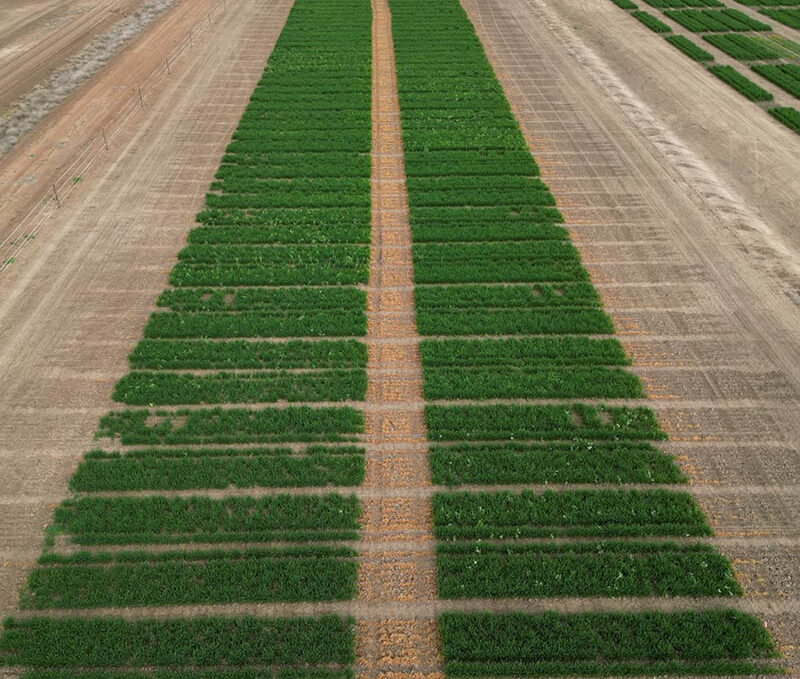
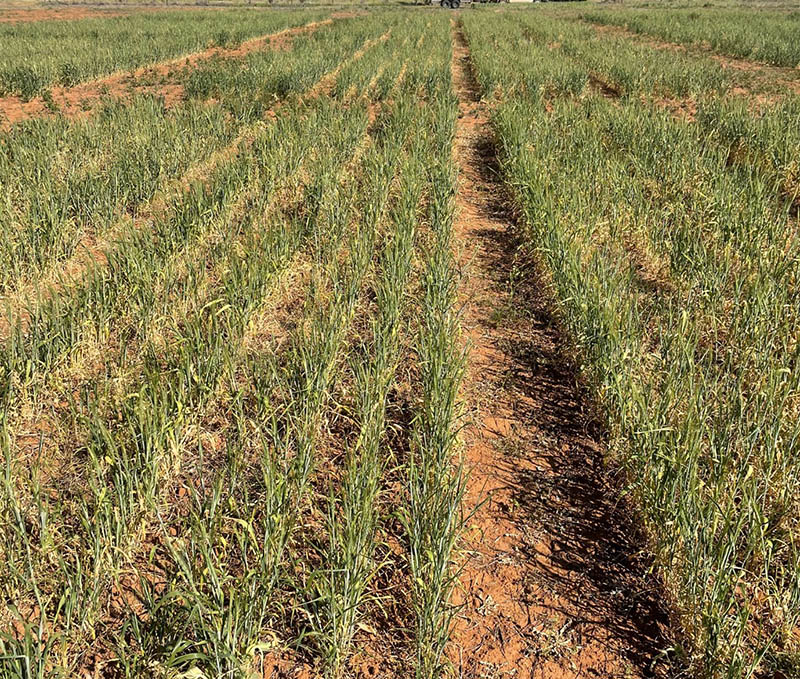
Summer cover crops
- Growing crops to provide living groundcover, as opposed to a stubble covered fallow, can build soil carbon in temperate systems of the northern hemisphere. To maximise potential soil benefits, it is often recommended a diverse mix is used, targeting different plant traits to enhance soil function. Examples include the use of legumes for nitrogen fixation and brassicas for soil conditioning.
- However, in Australia, water stored over the summer fallow period is important for winter crop growth, and cover crops may temporarily deplete soil nitrogen. While we have generally seen a decline in soil water at sowing with increasing summer cover biomass, good in-crop rain and nitrogen application has buffered against yield loss.
- Outside of these impacts, there is a major challenge to establishing summer cover crops in our climate. High evaporation rates and heat stress have restricted cover biomass in our trials to around one tonne per hectare. As a result, we have not seen an immediate impact on soil health indicators or soil carbon. Summer covers should therefore be viewed as an opportunistic practice to make use of summer moisture in wet seasons to provide a living cover.
Intercropping
- Temporary intercropping and synchronous intercropping are potential in-crop options for increasing plant diversity in our farming systems.
- Temporary intercropping consists of growing two or more species and then terminating one using selective herbicide, e.g. wheat and vetch.
- Synchronous intercropping grows two crops to harvest, e.g. canola and field pea.
- These systems aim to capture the nitrogen fixation benefits of the legume, while providing diverse root and litter inputs to the soil. Competition mechanisms and differences between the species of plants can lead to a system that produces higher biomass and yields.
- In the temporary intercrop treatments, little additional biomass was produced, but there was no negative impact on yields. Synchronous intercropping has been promising in adding additional and diverse biomass inputs.
Summary
- Building and maintaining soil carbon is an important issue in cropping systems.
- Additional biomass, managing soil constraints and system fertility are the key building blocks for carbon.
- Building carbon in summer cover crop, intercropping and temporary intercropping systems is likely to be a decadal process without a step change in biomass production, such as inclusion of a pasture phase.
- Diverse cropping systems have the potential to build carbon and demonstrate environmental stewardship.
- Practices that sequester carbon or promote soil health will become increasingly important for maintaining market access into the future.
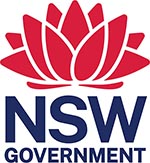
Prepared by the Soil CRC and their participants as part of the ‘Soil Carbon Capacity Building Resources for Farmers and Advisors’ project, proudly supported by NSW DCCEEW.
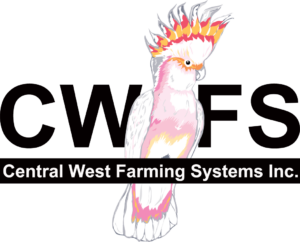